News
Author: KIT
During the third semester, researchers from KIT further studied and improved the conditions for the mechanochemical transformation of black mass (BM) into metallic black mass (MBM). Since BM supplied by ACC is already in a reduced state, they focused on reducing BM supplied by TES. This BM consists mostly of NMC (lithium nickel manganese cobalt oxides) cathode material and graphite, which was found to slow down the reaction kinetics. The reduction of the cathode active material by the metallic reducing agent result in the formation of the transition metals along with lithium oxide (Li2O) and the oxide of the respective reducing agent, which can be monitored by X-ray diffraction.
In contrast to the previous two semesters, researchers switched from shaker mills to planetary mills, which enable control of the rotation speed and larger volumes that can be processed. Various parameters such as ball-to-sample ratio (BSR), ball size, total load and rotation speed were investigated to optimise for a short reaction time.
Main take-aways
In general, the higher the BSR, the more mechanical energy can be transferred per gram of powder which results in a more intense milling and a faster reaction; however, this limits the throughput. Larger balls, on the one hand, lead to higher kinetic energies. On the other hand, fewer balls are used to keep the BSR constant resulting in a lower collision frequency. The maximum rotation speed is lower to prevent damage to the grinding media.
With Calcium as the reducing agent, no reaction was achieved at all. An unfavorable combination of ductility and size of the calcium pieces seems to resist further size reduction, which is required for the reaction.
Aluminium has the advantage of being used as a current collector and is already present in the black mass. However, during the reaction, LiAlO2 is formed, which is limiting the subsequent Li extraction efficiency in WP5. This problem can be avoided when magnesium is used as the reducing agent, which proved to be more reactive than aluminium but doesn’t form other lithium compounds than Li2O.
Compared to the shaker mill, a higher reaction rate was observed in the planetary mill. Researcher from KIT achieved a complete conversion of the lithium transition metal oxide in the planetary mill within 3 h using Mg as the reducing agent. In a larger version of the mill, the required milling time increases to 8 hours. Here, further investigations are planned for the next months.
Read previous article on the pre-treatment operations: Pre-treatment operations: Reactive milling for the production of metallic black mass
© Photo: Adobe
Author: KIT
Following previous work performed in work package 5, researchers from KIT further investigated the lithium (Li) extraction from black mass (BM) supplied by partners ACCUREC (ACC) and TES.
The BM provided by ACC consists of graphite, as well as transition metals such as nickel (Ni), manganese (Mn), and cobalt (Co), along with their respective oxides and impurities like copper (Cu), iron (Fe) and few fluorinated compounds. Li is present in the form of lithium carbonate (Li2CO3), lithium fluoride (LiF) and lithium aluminium oxide (LiAlO2). However, breaking off lithium aluminium oxide to a soluble Li-salt and removing fluoride contamination proved to be challenging.
Previous work reported the simultaneous incorporation of fluoride ions and decomposition of LiAlO2 using an excess of calcium hydroxide (Ca(OH)2) at elevated temperatures. More recent developments of the processes show improvement, especially decreasing considerably the amount of of Ca(OH)2 , while achieving a Li extraction of 87 %. Instead of heating the suspension, it is possible to initiate the reaction by liquid-assisted grinding in a planetary mill. The results show LiAlO2 is decomposed. However, the fluoride content is not efficiently removed.
In the BM supplied by the partner TES, most Li is present as lithium transition metal oxides (LiTMO) and a small amount of LiF. To enable Li extraction, this BM was reduced by reactive milling in Task 4.4 – Reactive milling for the production of metallic black mass (MBM). The obtained MBM consists of graphite, the metallic composite, lithium oxide and the oxidised reducing agent, along with some impurities like Fe and fluorinated compounds.
After investigating aluminium (Al) and calcium (Ca) in the previous steps, researchers at KIT performed tests to assess Li extraction using a magnesium (Mg) system, which presents various advantages, namely: avoiding, on one hand, the formation of Li salts with bad solubility, and relying on the other hand on insolubility of Mg in the high pH aqueous solution.
After aqueous extraction, researchers obtained a mixture of Li2CO3 and LiF. In the upcoming months, this mixture will be purified to a battery-grade material.
Authors: CHA and PNO
An important component of a Li-ion battery (LiB), the electrolyte has a crucial role in the cell performance. The nonaqueous electrolyte is a multicomponent system consisting of a conductive salt, mainly LiPF6 (Lithium hexafluorophosphate – inorganic component), organic carbonate solvents and additives.
Numerous research initiatives are dedicated to the design of the electrolyte composition, aiming to optimized performance, increased safety, lifetime and streamlined costs. However, once a LiB reaches its end-of-life (EoL) stages, the electrolyte receives less attention in the favour of the valuable metals that can be recycled from the cathode: Li, Co, Mn, Ni, Al, Cu. Moreover, due to its volatility, toxicity and high flammability, the electrolyte recycling is less studied. When ignored, the spent electrolyte reacts with water to form fluoride, leading to its uncontrolled decomposition and/or evaporation, and thus generating an imminent environmental risk. Due to its composition that includes organic solvents, the presence of residual electrolyte in the black mass is considered hazardous, and it is often a reason hampering the recycling process.
Researchers at Chalmers University (CHA) ) developed a sub- and supercritical carbon dioxide (sc-CO2) extraction process to selectively recover the electrolyte from spent LiBs. Sc-CO2 extraction technologies are already widely employed in the food, beverage, pharmaceutical, and cosmetic industry. In a nutshell, sc-CO2 forms when CO2 surpasses its critical point at 31°C and 73.8 bar pressure. In this so-called supercritical state, CO2 demonstrates optimal mass-transfer characteristics and can be fine-tuned to alter its physicochemical characteristics by adjusting pressure and/or temperature. However, CO2 proves ineffective as a solvent for high molecular weight polymers and highly polar ionic compounds. However, the addition of a co-solvent or a modifier can significantly improve the solubility properties of sc-CO2, making this alternative a suitable process to selectively extract LiPF6 after solvent removal.
Researchers at CHA investigated different process parameters (pressure, temperature, extraction times) to understand their impact on the extraction behavior of different electrolyte solvents such as ethylene carbonate. Their analysis included both qualitative and quantitative results indicating the composition and the purity. Simultaneously, the research also monitored the process exhaust stream for potential formation of LiPF6 decomposition products. The findings showed that that the non-polar electrolyte solvents like dimethyl carbonate, diethyl carbonate, and ethyl methyl carbonate were successfully extracted using low-density CO2. The more polar electrolyte components such as ethylene carbonate and propylene carbonate were stepwise extracted by gradually increasing the system’s pressure. The developed technology is a game changer not only for electrolyte recycling but also for increased workplace and transportation safety due to removal of the flammable and hazardous substances from battery black mass. The simplicity of the processing design is an additional advantage of the technology.
© Photo credit: Adobe
The modified Black Mass (BM) obtained in Task 4.4 – Reactive milling for the production of metallic black mass (MBM), as well as the BM supplied by partner ACCUREC (ACC) were leached under different conditions to extract lithium (Li) salt.
The BM provided by ACC consists of graphite, as well as transition metals such as nickel (Ni), manganese (Mn), cobalt (Co), along with their respective oxides and impurities – copper (Cu), iron (Fe), and few fluorinated compounds. Li is present in the form of lithium carbonate [Li2CO3] and lithium aluminium oxide [LiAlO2 ]. However, breaking off LiAlO2 to a soluble Li-salt and removing fluoride contamination is a challenging process. To overcome this, the BM was heated with calcium hydroxide in deionised water for one hour and then filtered.
Illustrated in the next figure, LiAlO2 decomposes during heating and transforms into insoluble calcium aluminium hydroxide. Dissolved fluoride anions are caught by Ca2+ ions and precipitate as calcium fluoride. The soluble part only contains Li- and Ca-salts. The latter can be transformed into calcium carbonate and removed with a second filtration step.
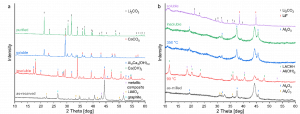
X-ray diffraction patterns of leaching and purification process. a) black mass supplied by ACC and treated with calcium hydroxide. b) aluminium system of modified black mass
The second BM from partner TES, obtained after ball milling, consists of graphite, the metallic composite, lithium oxide and the oxidized reducing agent, along with some impurities like Fe and fluorinated compounds.
Lithium extraction after ball milling
In the aluminium system, the extraction of lithium salt poses certain challenges. Due to the tendency of residual aluminium powder to ignite when in contact with air, this requires cautious handling. During milling lithium aluminium oxide is formed, and in the basic solution, a significant amount of aluminium hydroxide dissolves and reacts with lithium hydroxide and CO2, resulting in the formation of a poorly soluble compound called LACHH. To address this problem, the suspension is heated to 90°C to reinforce the reaction between aluminium hydroxide and lithium hydroxide. The LACHH formed can be decomposed at 350°C into insoluble aluminium oxide and soluble lithium carbonate, which can be separated through filtration.
Lithium extraction in the calcium system, on the other hand, is a relatively straightforward process. The milled powder shows low reactivity towards both oxygen and water. Scientists successfully extracted 98% of lithium from the black mass; however, calcium hydroxide was also dissolved in the process. Subsequent purification steps led to the isolation of lithium carbonate with a purity of 93% in a yield of 75%. The main impurities are shown in the figure displayed below.
In the upcoming months, researchers will work to improve the leaching conditions towards a lower reagent and water consumption, while obtaining a higher purity of resulting lithium salt and lower lithium loses during purification steps.

Impurity cations in isolated lithium carbonate from the calcium system of modified black mass
During the second semester, researchers from KIT further studied and improved the conditions for the mechanochemical transformation of Black Mass (BM). With the BM supplied by ACC is already in a reduced state, the focus now shifts towards reducing the BM provided by partner TES.
This BM consists mostly of nickel-manganese-cobalt (NMC) cathode material and graphite, and it was found to cause a longer reaction time. It is expected that the graphite exfoliates during milling and creates thin protective layers around NMC particles and the reducing agent, slowing down the reaction kinetics.
Using variations of milling parameters like ball-to-sample ratio or the type and amount of reducing agent, researchers optimised the process towards the fastest kinetics. During the investigation, no intermediate reduction to transition metal oxides in lower oxidation states was observed. However, the full reduction of the respective part to the metallic composite occurred.
The operation took place in a shaker mill, and it requested an excess of 3.3 equivalents of Aluminium (Al) towards NMC was required to attain a complete reduction within a reasonable time. This transformation was accompanied by the formation of aluminium carbide and the presence of residual fine aluminium powder which caused a self-ignition hazard when the milled powder came into contact with air.
In contrast, when using calcium as a reactive agent, researchers observed a fast reaction but they also reported a strong dependency of reaction kinetics on calcium size. The hazard of self-ignition when exposed to air was limited in this case by using stoichiometric amounts.
In addition, the research team has been conducting preliminary experiments for the scale-up process using a planetary mill. It was observed that the choice of the milling type has a significant impact on the reaction kinetics. Attempts to crush calcium pieces proved to be unsuccessful, therefore not initiating any reaction. However, when using aluminium, reactions occurred much faster.
In the months ahead, the research team working in WP4 will continue to investigate the milling parameters for the planetary mill.
Find out more information about the activities developed in WP4 in the article Reactive milling for the production of metallic black mass (MBM) – Rhinoceros project (rhinoceros-project.eu)
Read more about the black mass leaching operations in the article Materials extraction and direct routes for the synthesis of electrode materials: Recovery of Lithium as battery grade materials
Focusing on mechanochemical (MC) processing, the chemical transformation of the black masses (BMs) supplied by partners ACCUREC and TES, is planned to be carried out within Work package 4.
Before MC processing, the black masses were analysed using a combination of different analytical techniques. Both quantitative and qualitative analysis were undertaken to determine the Lithium and transition metals yield of the developing recycling process.
Using different reducing agents such as Al, Ca, and their mixtures, researchers carried out preliminary investigations of the MC processing of BMs. Within this task, different aspects, such as the role of the ball milling conditions, the ball milling time, presence of other nonreactive components, and nature of the reducing material were investigated. The analysis led to the conclusion that the kinetics of the MC-induced reduction reaction is sensitive to multiple processing parameters, as shown in the featured image:
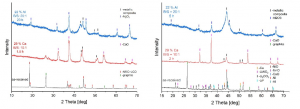
XRD patterns of the as-received BMs and products of their reduction after MC processing with Al and Ca as reducing agents: left-TES material; right-ACC material
The upcoming research will focus on improving the reduction reaction kinetics and eliminating the possible safety hazards of fine powder materials. Once finalised, this work will determine the optimal ball milling conditions to be scaled up.
Recovery of Lithium as battery grade materials
The process described in Task 4.4 leads to the chemical transformation of the black masses (BMs) into ferromagnetic Co-, Ni- and Mn-containing products, which will be separated from other by-products. Lithium will be extracted from the rest of the solid products in the subsequent aqueous leaching process to be further transformed into battery-grade lithium carbonate (Li2CO3) salt.
Within the M1-M6 period, aqueous leaching of the ball-milled samples using Al and Ca as reducing agents (RA) was carried out. At a preliminary stage of investigations, researchers noticed the resulted Li2CO3 materials presented small amount of impurities.
To increase the yield of lithium recycling, in the upcoming period, the research work will target the improvement of the leaching conditions and the purification process.
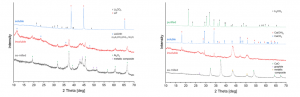
X-ray diffraction patterns of the as-milled BM obtained from TES, products of their reduction reactions after MC processing with Al (left) and Ca (right) as RA, and obtained Li salts after aqueous leaching
TECNALIA [TEC] has actively undertaken both the coordination tasks and the experimental activities that correspond to the solvometallurgical treatment of the received black masses. Firstly, the coordination of the project started with the preparation of the kick-off meeting, in which the entire Consortium assembled in San Sebastián (Basque Country – Spain) and comprises administration and management to ensure an efficient development of RHINOCEROS project.
Also, the experimental section concerning the critical materials extraction from the received black masses from spent batteries started out after receiving the samples from partners ACCUREC [ACC] and KIT.
After characterisation, TEC performed a first round of tests to these samples using a solvometallurgical route and assessing pre-treatment effect on the process. In parallel, State of Art is analysed for different relevant solvometallurgical systems aiming lithium recovery. New batches of experiments will be performed for process optimisation and new tests will also be performed when further black mass samples are received.